Our laboratories offer excellent working conditions and are equipped with the-state-of-the art equipment and instrumentation. Here follows more information on the specific instruments used for the various types of analysis and characterization.
Laboratories at the Physics department, NTNU (Erika Eiser)
In 2022 Professor Erika Eiser set up her experimental lab dedicated to the development and study of bio-inspired, sustainable materials.
Contact: Erika Eiser
Studying the structure of nanoporous networks and transport/flow through them experimentally requires both a wet lab for the preparation of those and a dry lab equipped with appropriate instruments such as microscopes with fast cameras and micro-rheology tools.
The wet lab was established to synthesize and functionalize various colloids. Amongst those are fluorinated latex particles that form colloidal crystals with tunable structural colors1. The Eiser Group also studies DNA-functionalized colloidal networks with well-defined pore-size distribution. Here, a dense brush of short single-stranded DNA attached to the colloidal surfaces acts as a temperature induced ON-OFF switch for very strong, short-ranged attraction. The selectivity of DNA-binding enables the formation of two intertwined, percolating networks called bigels 2,3 (Fig. Wet Lab). Moreover, our colloids can be used to induce out-of-equilibrium crystallization at liquid-liquid interfaces4.
Further, we use a bio-mimetic approach to develop clay-biopolymer nanocomposite films and coatings made from Laponite, a synthetic clay, and cetylmethyl cellulose5
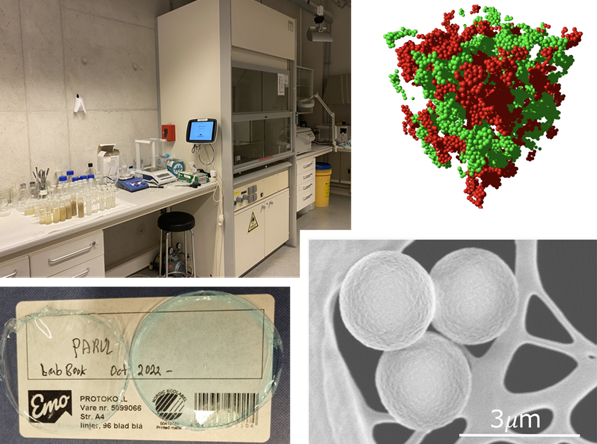
The dry lab was developed to focus on the study of the rheology of complex fluids and eventually two-phase flow in porous media. Over the past 15 years the Eiser group has explored various light scattering based particle sizing and micro-rheology techniques to probe the viscoelastic equilibrium properties of complex systems. Amongst those techniques are Differential Dynamic Microscopy (DDM)6 that allows the determination of particle sizes between 50 nm and several micrometer using simple video-microscopy. This technique proved particularly useful to study the diffusivity of colloids in confining porous networks7.
When working with precious samples such as protein solutions or DNA hydrogels, it is imperative to use micro-rheology rather than classical bulk rheology. We use Dynamic Light Scattering (DLS)8 and Diffuse Wave Spectroscopy (DWS) based micro-rheology9 as well as a dual Optical Tweezers setup (Fig. Dry Lab). In all three cases we measure the thermal fluctuations of the inserted probe colloids to obtain their mean-squared displacement <Dx(w)2>, which is directly related to the elastic and loss modulus, G’(w) and G’’(w), of the background fluid. The advantage of these methods is that we can measure the local and bulk viscoelastic response of highly complex fluids, which cannot be achieved with conventional methods. Moreover, they allow us to explore the systems response over a large temperature range. Both DWS and DLS measurements are fast, allowing also for time-resolved experiments.
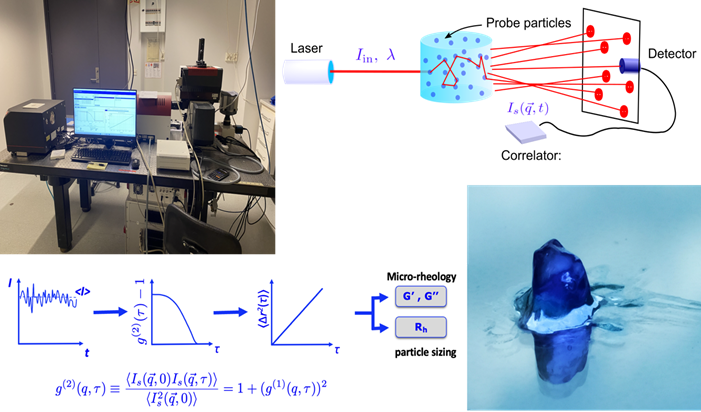
References:
- T. Erdem, T. O’Neill, M. Zupkauskas, A. Caciagli, P. Xu, Y. Lan, P. Bösecke, E. Eiser ´Transparent Colloidal Crystals With Structural Colours´ Frontiers in Physics, Sec. Soft Matter Physics, 166 (2022)
- F. Varrato, L. Di Michele, M. Belushkin, N. Dorsaz, S.H. Nathan, E. Eiser, G. Foffi, “Arrested demixing: from gels to bigels”, PNAS 109 (47), 19155 (2012)
- L. Di Michele, F. Varrato, J. Kotar, S.H. Nathan, G. Foffi, E. Eiser, “Multistep kinetic self-assembly of DNA-coated colloids”, Nature Comms, 4:2007 (2013)
- A. Caciagli, R. Singh, D. Joshi, R. Adhikari, E. Eiser ‘Crystallization of interfacially tethered colloids in an emergent optofluidic potential’, Phys. Rev. Lett. 125, 068001 (2020)
- P. Xu, T. Erdem, E. Eiser “A Facile Approach to Prepare Self-Assembled, Nacre-Inspired Clay/Polymer Nano-Composites”, Soft Matter 16, 3385 (2020)
- E. Eiser, “Chapter 5: Dynamic Light Scattering” in Multi Length-Scale Characterisation, Wiley, .ch5, 233 (2013)
- M. Zupkauskas, Y. Lan, D. Joshi, Z. Ruff & E. Eiser, “Optically Transparent Dense Colloidal Gels”, Chemical Sciences, 8, 5559 (2017)
- R. Liu, A. Caciagli, J. Yu, X. Tang, R. Ghosh, E. Eiser ´ Dynamic Light Scattering based microrheology of End-functionalised triblock copolymer solutions´ Polymers 15 (3), 481 (2023)
- 26. Z. Xing, A.Caciagli, T. Cao, I. Stoev, M. Zupkauskas, T. O’Neill, T. Wenzel, R. Lamboll, D. Liu, E. Eiser “Microrheology of DNA-Hydrogels” PNAS 115 (32), 8137 (2018)
- I. D. Stoev, A. Caciagli, Z. Xing, E. Eiser “Using Single-Beam Optical Tweezers for the Passive Microrheology of Complex Fluids” SPIE – Proceedings of Optical Trapping and Optical Micromanipulation XV, 107232D (2018)
Selected Laboratory setups of the X-ray physics group, department of Physics, NTNU (Dag Breiby)
The X-ray physics group is Norway’s leading research unit in X-ray physics and imaging.
Time-resolved computed tomography for flow in porous media
Time-resolved computed tomography (CT) allows studying single- or multiphase flow in porous media. By injecting fluids into a porous sample, while at the same time performing CT measurements, the flow dynamics can be resolved in 3D. To obtain time-resolved 3D images, reconstruction methods requiring few measured projections are required. Here, compressed sensing algorithms are used, which enable tomography with a time resolution as good as 30 seconds. The time-resolved 3D maps can be analyzed to provide a better understanding of multi-phase flow in porous media, which is important, for example, for improving carbon capture and storage (CCS) technology.
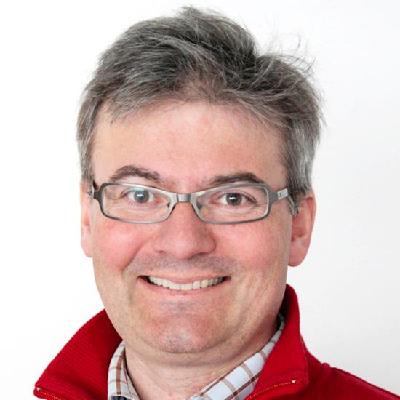
Small- and wide-angle X-ray scattering
Contact: Dag W. Breiby
X-ray scattering can be used to identify e.g. particle sizes, sample compositions and crystal lattice parameters. Our in-house equipment can be used for both small-angle X-ray scattering (SAXS) and wide-angle X-ray scattering (WAXS), allowing structural features of different sizes to be studied. SAXS/WAXS can be done on several sample types, ranging from particles dispersed in solutions, to centimeter-sized bulk samples. By translating the samples in x and y (see Fig. 2), spatially resolved maps can be obtained, and the two-dimensional (2D) detector, facilitates information about the structure orientation to be captured.
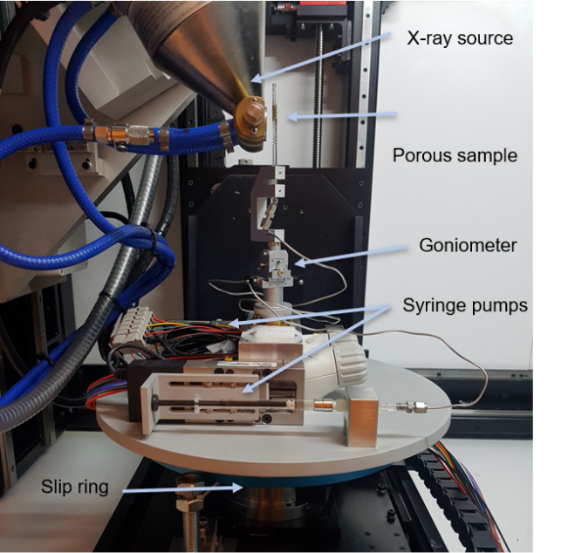
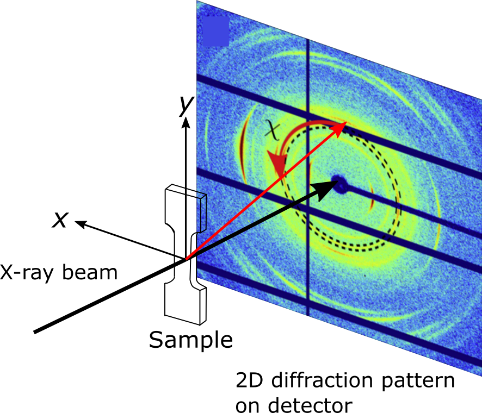
Fourier ptychography microscopy
Fourier ptychography microscopy (FPM) is a recent optical microscopy technique for quantitative imaging of samples with a large field of view. The recovered quantitative amplitude and phase maps can be used to study porous media and biological materials. Figure 3 shows the in-house FPM setup. The sample is sequentially illuminated by partially coherent light-emitting diodes (LEDs) in a 2D array, and the transmitted light is focused by an objective lens onto a camera. As each LED illuminates the sample from a unique direction, the recorded transmission images can be numerically combined to give high-resolution quantitative amplitude and phase images. The quantitative phase images are useful for studying weakly absorbing samples, such as biological tissue, or to study fractures in glass surfaces. Figure 4a shows the reconstructed quantitative phase image from a piglet cartilage histology sample. The quantitative phase information can be used to calculate scattering coefficients (Fig. 4b) giving information about the light transport through the sample.
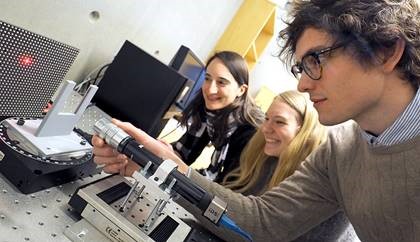
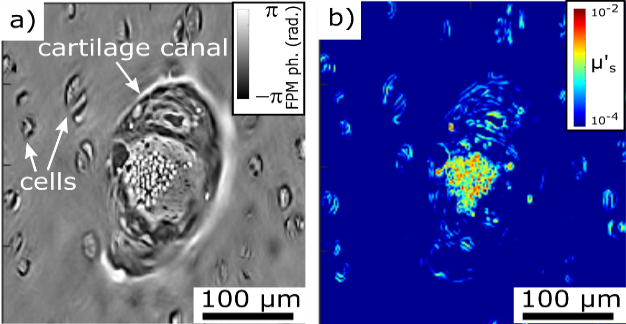
Laboratories at the Physics Department, University of Oslo
PoreLab has four specialized laboratories at UiO, all of which are equipped with a wide set of state-of-art techniques to study the dynamics and structure of flow in 2D and 3D porous media.
The laboratories have a full range of high-resolution and high-speed imaging techniques, including two ultrafast Photron Ultima (SA5 and APX) cameras with 7000 fps. at a spatial resolution of 1024×1024 pixels and up to 1 million fps. at a reduced resolution.
In 2020, an important part of our activity has been 3D modeling. PoreLab has acquired a Carbide Shapeoko XL CNC milling machine and two Formlabs Form 3 3D printers that are based on a new Low Force Stereolithography (LFS) technology. This technology allows for 3D printing of very fine, high resolution models in a variety of resin types. The acquisition of these state-of-the-art printers gives us a unique opportunity to quickly design and 3D print synthetic porous materials. Apart of the foray into 3D modeling, we have also engaged in improving the imaging equipment in the labs. The latest addition to our wide array of different cameras are two new high-speed cameras, the Photron WX100 mini. These new cameras can take 4 MP images at 1000 fps (FullHD at 2000 fps) and all the way up to 80K fps for lower resolutions.
PoreLab has also a high-resolution FLIR SC300 infrared camera used for real-time measurements of heat dissipation in fractures, hydro-fractures and porous media flows and a wide variety of DSLR cameras and accompanying optics.
Microscale experiments can be imaged via far field microscopy using a Zeiss Stemi 2000-C distortion-free stereomicroscope that couples to our high-speed and high-resolution cameras. This is currently in process of being upgraded for enhanced magnification. Flicker-free illumination sources tailored for the different applications (including high-speed microscopy) are also available. PoreLab have recently bought a Krüss DSA25 drop shape analyzer to perform direct measurements of surface tension, wetting properties and surface free energy, as illustrated in Fig.1.
Additionally, the PoreLab laboratories include a large set of different optical equipment, such as lasers with different intensities and wavelengths, lenses and other optical components, cameras and microscopes for Particle Image Velocimetry. The labs are well equipped to perform homodyne correlation spectroscopy for the measurement of particle velocity fluctuations in fluids, diffusion constants and viscosities. PoreLab developed a 3D optical scanner which makes it possible to measure 3D fluid structures in refraction index matched porous media. This equipment can be used to study dispersion in mono-phase flow and two-phase flow studies.
In addition to this wide variety of state-of-art techniques, our laboratories are also fully equipped with standard fluid mechanics labware, such as capillary viscometers, high-precision scales, pressure and temperature sensors, surface treatment chemicals for the control of wetting properties and general laboratorial glassware. By focusing on both standard and state-of-art techniques in the same physical space, our laboratory benefits from a relatively high degree of independence.
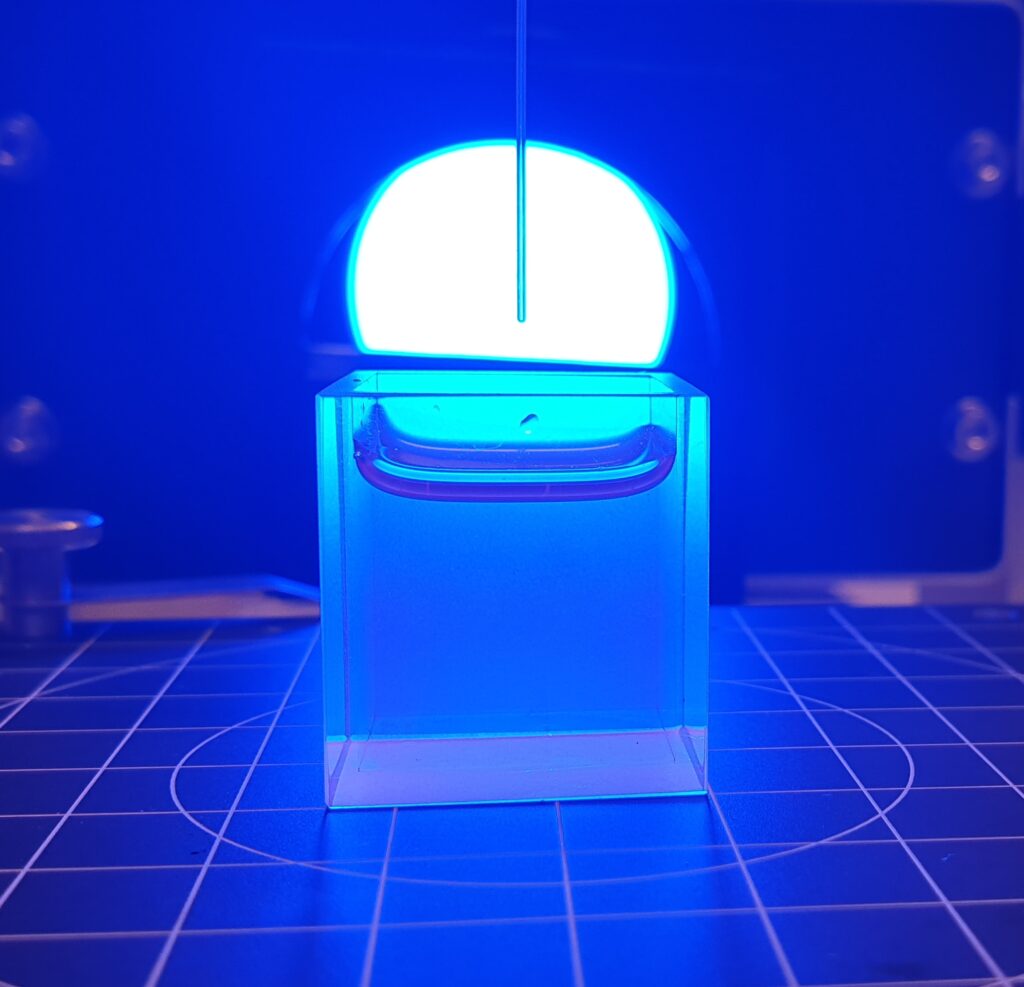
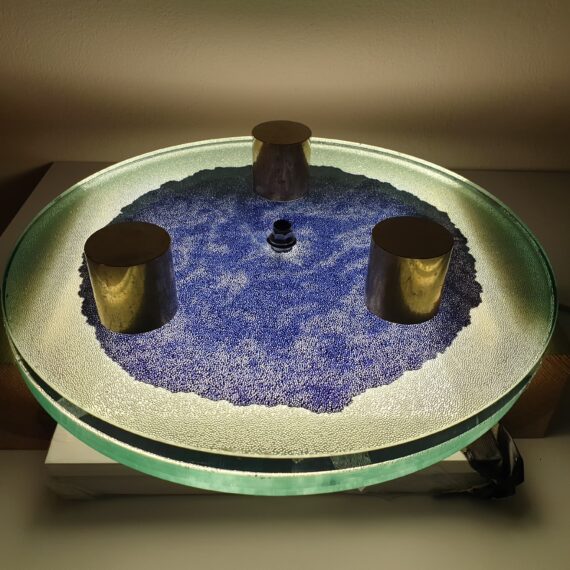
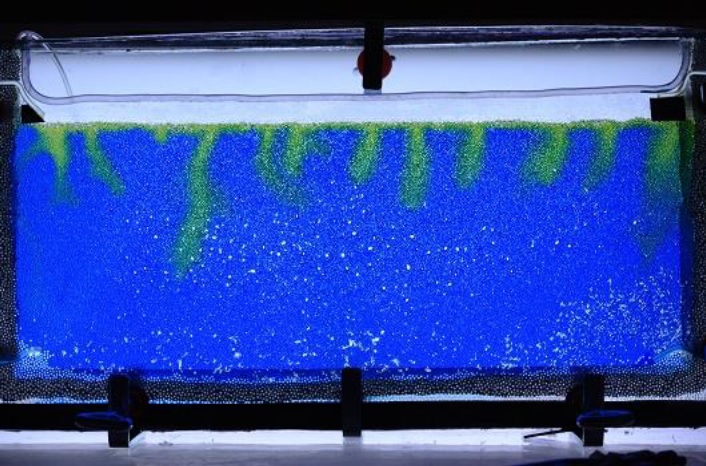
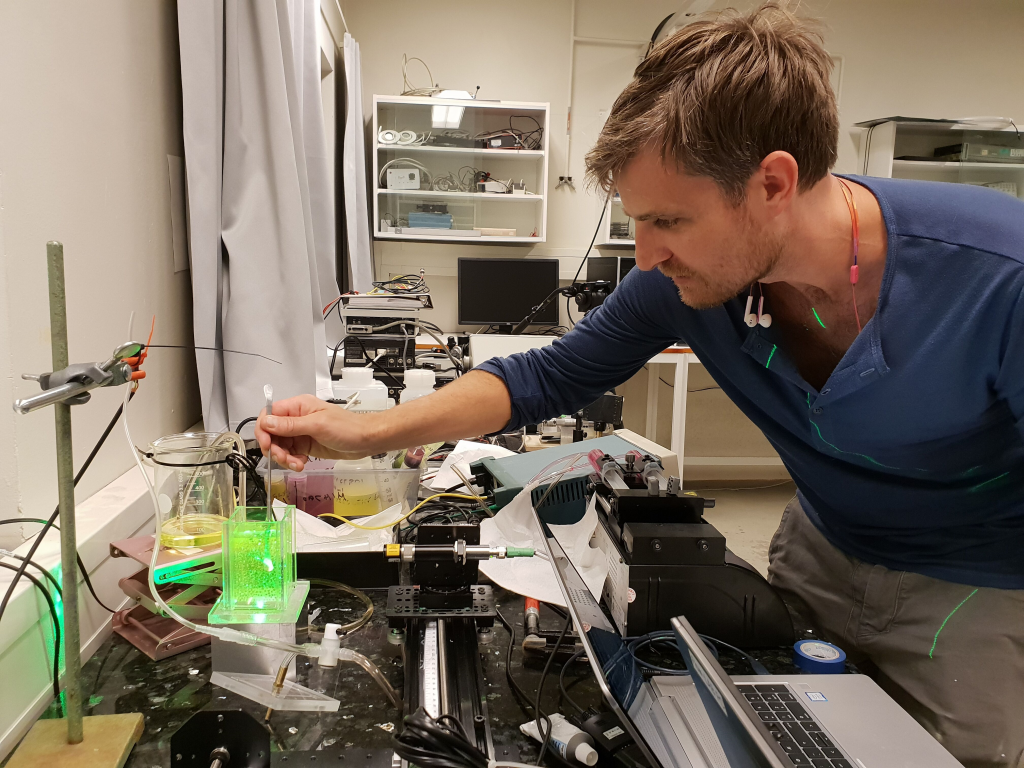
In a continuous effort to improve our experimental toolbox, we are constantly investigating possibilities of new technologies for fabricating quasi 2-D and 3D porous models suitable for different experimental needs. Those models are at the core of a good fraction of our experimental activity in porous media flows. Glass beads models, which have become our labs signature approach in this type of research, are now complemented with 3D printed models based on a Low Force Stereolithography technology. Since 2020, we have been able to successfully employ this 3D printing technique in a number of different projects. In 2021 we received a brand new Formlabs Form 3L printer having a five times larger build volume than its predecessor. The Formlabs Form 3L printer is the newest addition to Formlabs portfolio and to our labs. We were lucky enough to get one of the first five printers that came to Norway after their release in 2020 and this printer is now at full operation in our labs at the University of Oslo. Apart from being able to print incredibly high-quality parts with horizontal resolution down to 25 micrometers, the newest addition to our printer farm (currently 3 SLA printers) is also able to print in a variety of functional resins.
3D printing the porous networks opens a new avenue of experimental research in the field: we can now directly control the porosity and permeability of our systems, and even design configurations of immediate practical relevance, like layered media or systems with dual porosity. These investigations were previously only accessible numerically, so we are very enthusiastic to bring now the much-needed experimental validation to these works. Having several printers at our disposal allows for faster prototyping and parallel production of the models. This in practice makes the experimental workflow much smoother and potential issues can be addressed in a faster way. All this being said, our legacy glass beads model, something that our group built its status and name on are still going to be used in scenarios where they are more appropriate.
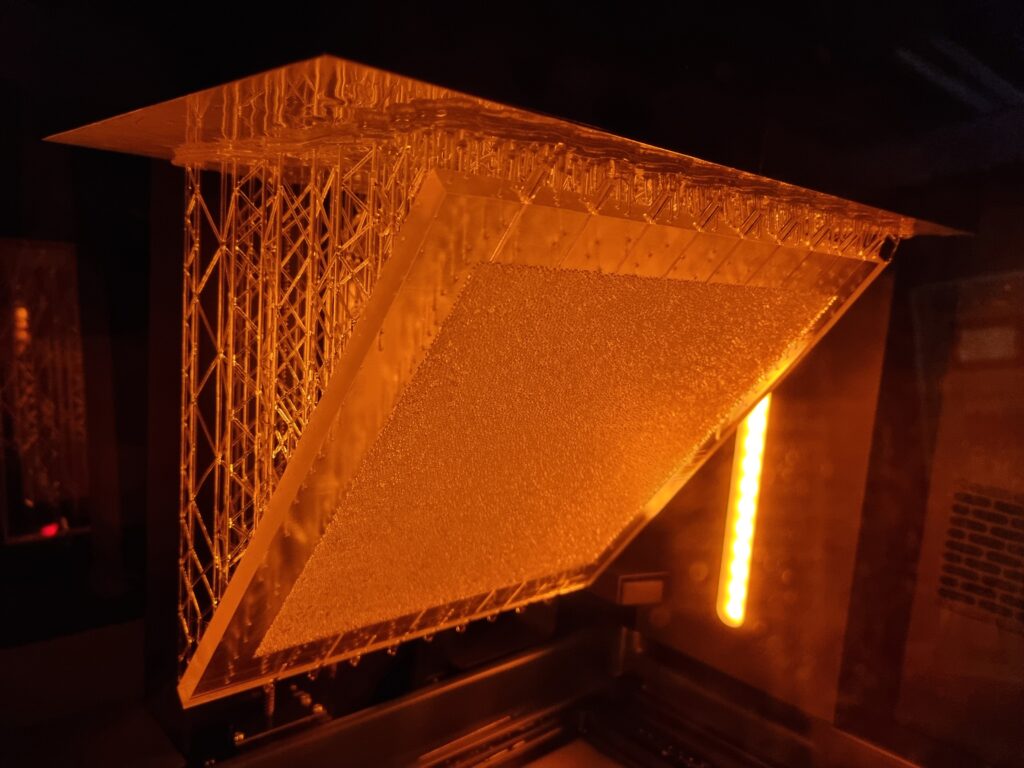
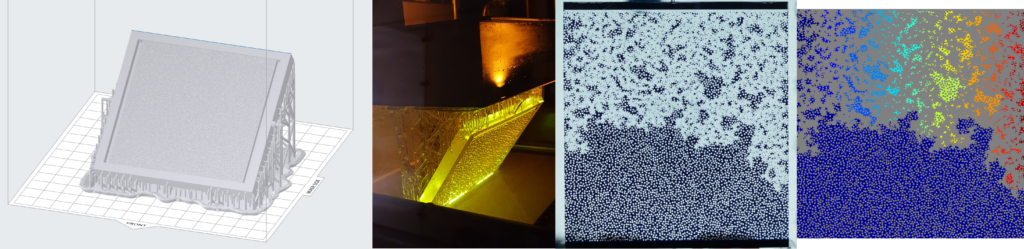
Laboratories at the department of Geoscience and Petroleum, NTNU
A NEW CT-SCANNER AT THE DEPARTMENT OF GEOSCIENCE AND PETROLEUM, NTNU
On October 29th, 2021, was organized the ribbon-cutting ceremony for the new CT-scanner at the department of Geosciences and Petroleum, NTNU. The new scanner was named “Ole” after Professor Ole Torsæter who had been a mentor and a driving force for the acquisition of this up-to-date equipment. Ole Torsæter has been the Principal Investigator for the Work Package related to Microfluidics and Field Studies at PoreLab until he retired in November 2019. His successor is Associate Professor Carl Fredrik Berg. Ole is now Professor Emeritus and still very much involved in PoreLab’s activities.
The new CT-scanner is part of the equipment financed by the Research Council of Norway under PoreLab’s umbrella.
Post-doctoral researcher, Haili Long-Sanouiller explains that: “Detailed capture and measurement of internal component and assembly features is often vital for material and porous media research, quality control and failure analysis. The Nikon, XT H 225 system offers a microfocus X-ray source, an inspection volume to accommodate small to medium sized parts and high image resolution in 3D. Ready for ultrafast CT reconstruction, the XT H 225 covers a wide range of applications, including the inspection of pore spaces, plastic parts, small castings and complex mechanisms as well as researching materials and natural specimens.” The same day, on October 29th, the department of Geosciences and Petroleum was also celebrating the 70th anniversary for Ole Torsæter. In addition to the ribbon-cutting ceremony for the new CT-scanner, the day was marked with flowers, cakes and speeches.
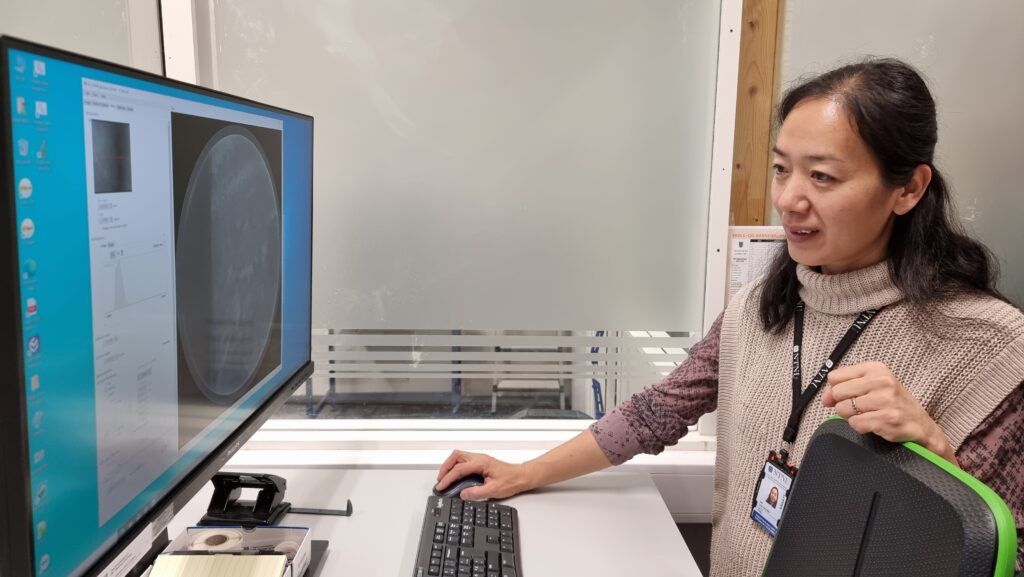
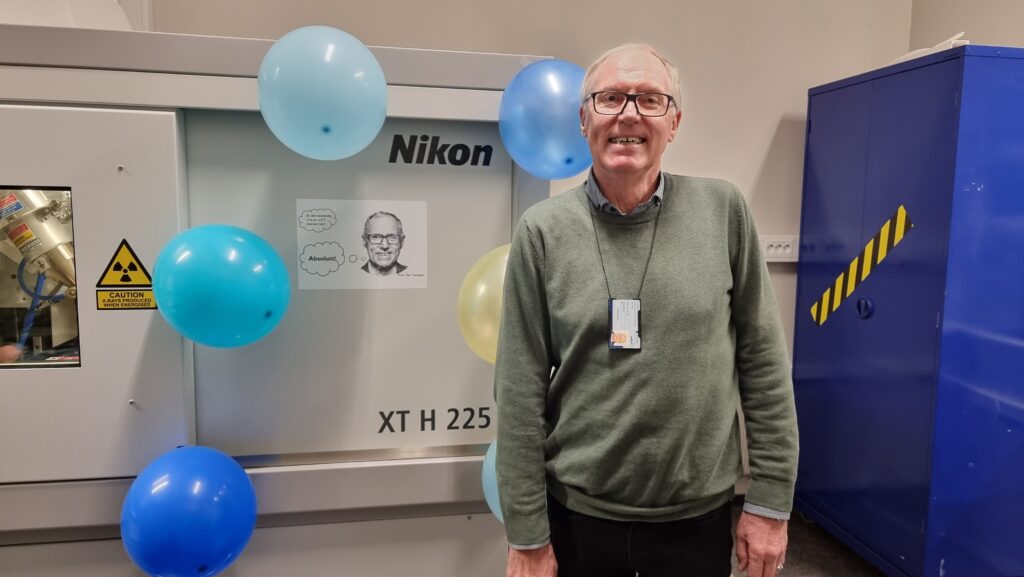
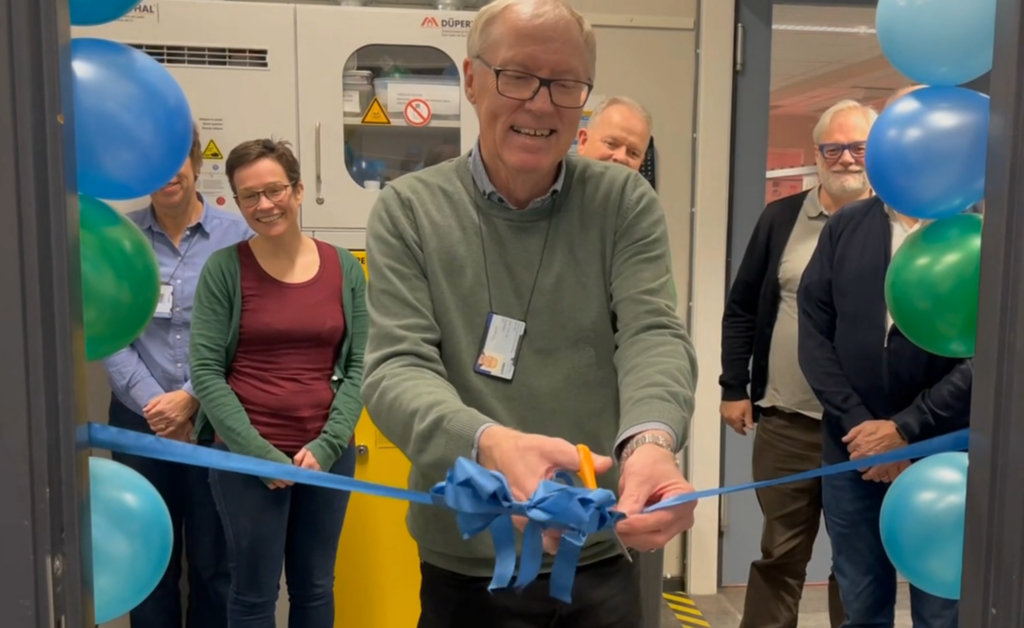
The core analysis laboratory is equipped with state-of-the-art equipment for routine and special core analysis. Included are core preparation equipment (drilling, cutting, cleaning and saturating core plugs), porosimeters, permeameters and apparatus for core plug resistivity measurements. The laboratory has specialized equipment like automated centrifuge for capillary pressure and relative permeability measurements and core flooding rigs for various enhanced oil recovery processes. The main components in the flooding rigs are core holders, pumps, fluid lines, fluid containers, pressure sensors and flow meters.
The laboratory is also equipped with micro-computer tomograph (CT). This micro-CT has a tailor made miniature (5mm diameter) core flooding set up which is used in pore scale studies. In addition the laboratory has microfluidics apparatus where the main components are glass micro models, syringe pumps, microscopes and digital cameras with large monitors.
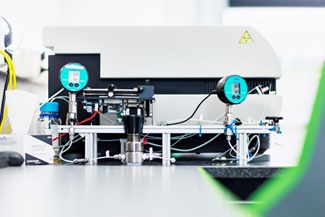
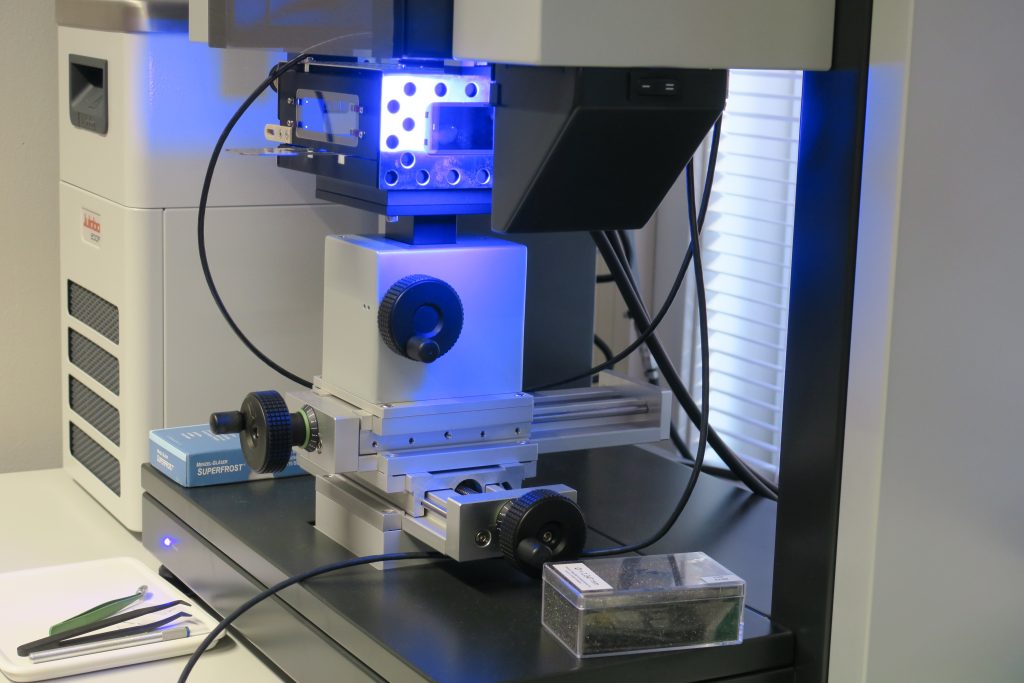
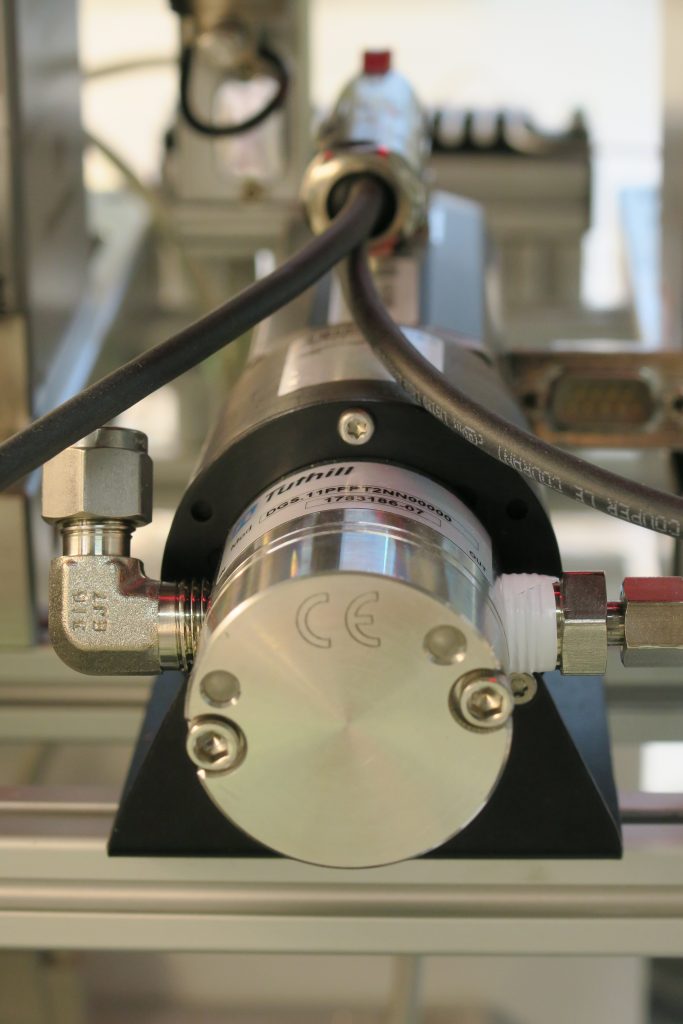
A description of the main equipments
Supplementary equipment for fluid and fluid/solid interaction analysis includes interfacial tension apparatus (pendant drop and spinning drop), contact angle apparatus, devices for zeta potential and particle size measurements, densitometers, viscosimeters and rheometers.