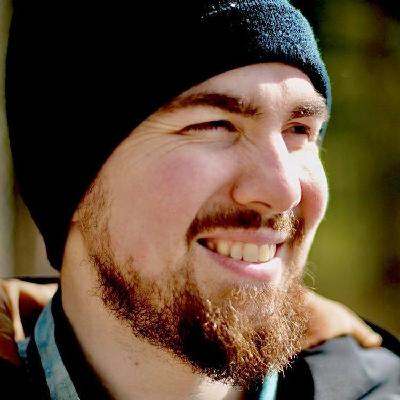
PHD TRIAL LECTURE AND PUBLIC DEFENCE
KIM ROGER KRISTIANSEN– THE DEPARTMENT OF CHEMISTRY
Kim Roger Kristiansen has submitted the following academic thesis as part of the doctoral work at the Norwegian University of Science and Technology (NTNU):
Thermal Driving forces Across Membrane Systems: Energy Conversion and Water Purification
Assessment Committee
The Faculty of Natural Sciences has appointed the following Assessment Committee to assess the thesis:
- Professor Fernando Bresme, Imperial college, UK
- Senior scientist Torleif Holt, SINTEF, Norway
- Professor Carlos Dorao, Department of Energy and Process Engineering, NTNU
Professor Carlos Dorao has been appointed Administrator of the Committee. The Committee recommends that the thesis is worthy of being publicly defended for the PhD degree.
Supervisors
The doctoral work has been carried out at the Department of Chemistry, where Professor Signe Kjelstrup has been the candidate’s supervisor. Professor Knut Jørgen Måløy has been the candidate’s co-supervisor.
Public trial lecture:
Time: 31sth of May at 10:15
Place: S4, Sentral Building 1, NTNU Gløshaugen
Prescribed subject: Thermodynamic models for super hydrophobic surfaces considering both roughness and heterogeneous composition effects
Public defence of the thesis
Time: 31sth of May at 13:15
Place: S4, Sentral Building 1, NTNU Gløshaugen
Summary of thesis
Among the energy sources used to fuel our modern industrialized society, industrial waste heat is among the most abundant and untapped. Wherever energy conversion from high-grade sources occurs, a fraction of this energy is lost in the form of low-grade heat energy. More than half of this heat energy is dissipated in media at temperatures below the atmospheric boiling point of water, where operation of traditional energy conversion methods is limited. In this work, we have focused on the application of thermal driving forces in membrane systems designed to convert low-grade heat energy into high-grade energy and to produce potable water in order to address the increasing scarcity of both high-grade energy and potable water. We have studied two different types of membrane system for this purpose: one is designed to simultaneously produce potable water and mechanical energy, while another is designed to convert heat into electrical energy.
In our studies on the pressure-retarded membrane distillation (PRMD) process, most of the work revolved around the formulation of a non-equilibrium thermodynamics approach to generalize the already-existing models found in the membrane distillation (MD) literature. The rationale behind this was that membranes required for a feasible PRMD process would require conditions where effects not captured by traditional models may be important. By means of kinetic theory, a model was formulated to capture effects that cannot be neglected as membrane pores approach the nanometer scale. The findings in this work were combined with the development of a systematic non-equilibrium thermodynamics approach to decompose the transport coefficients of the membrane system on a macroscopic scale. In this framework, connections were made from physical models on the microscopic scale to measurable effects on the macroscopic scale. By means of appropriate approximations, we showed correspondence to the traditional MD models and demonstrated conditions under which the additional effects captured by the systematic non-equilibrium thermodynamics approach would be significant. Such conditions include nanoscale pore sizes and superhydrophobic membrane surfaces, both conditions beneficial for the performance of the PRMD process. A flow cell system was designed and built in order to obtain accurate flow measurements in response to tightly controlled temperatures and pressures across membranes. The apparatus was successfully commissioned with isobaric MD measurements, and measurements on the pressure-retarded process is planned as future work. Based on data from these measurements, further predictions were made on the potential of the PRMD process. It was found that the mechanical power density of PRMD could well approach values found in commercial pressure-retarded osmosis (PRO) if membranes are properly designed specifically for high PRMD performance. We highlight that the PRMD process has potable water as byproduct, and the pressure can be adjusted to regulate the balance between the production of mechanical energy and potable water.
We also studied the potential for conversion of heat to electrical energy by means of thermogalvanic cells. We started the work by designing a flow cell to provide accurate measurements of the Seebeck coefficients of ion-exchange membranes. The experimental work combined with the theoretical developments allowed us to demonstrate a systematic approach to describe the coupled transport phenomena. A review paper was published where several results from the literature were all interpreted within the same theoretical framework. We concluded that more systematic work is needed to correlate the structural and chemical properties of ion-exchange membranes to their performance as components in thermogalvanic cells. To ensure that such studies are well-designed and that the results are correctly interpreted, the theoretical framework and conventions in the field need to be standardized. A perspective article was formulated as an attempt to set the record straight on the connections between different conventions used within non-equilibrium thermodynamics descriptions of thermogalvanic systems. We highlighted potential pitfalls where one would interpret a measured quantities to relate to purely theoretical non-measurables, and made recommendations for a standardized approach that makes only reference to measurable quantities.
This work has contributed towards clarifying and standardizing theoretical descriptions of non-isothermal membrane systems, and provided new insights that can help direct the development of membrane systems both for the PRMD process and for thermogalvanic systems such as non-isothermal reverse electrodialysis. The methodology is presented in a transparent manner intended to provide the reader with the insight required to apply the same methodology to systems not specifically investigated in this work.