VILDE BRÅTEN – DEPARTMENT OF MATERIALS SCIENCE AND ENGINEERING
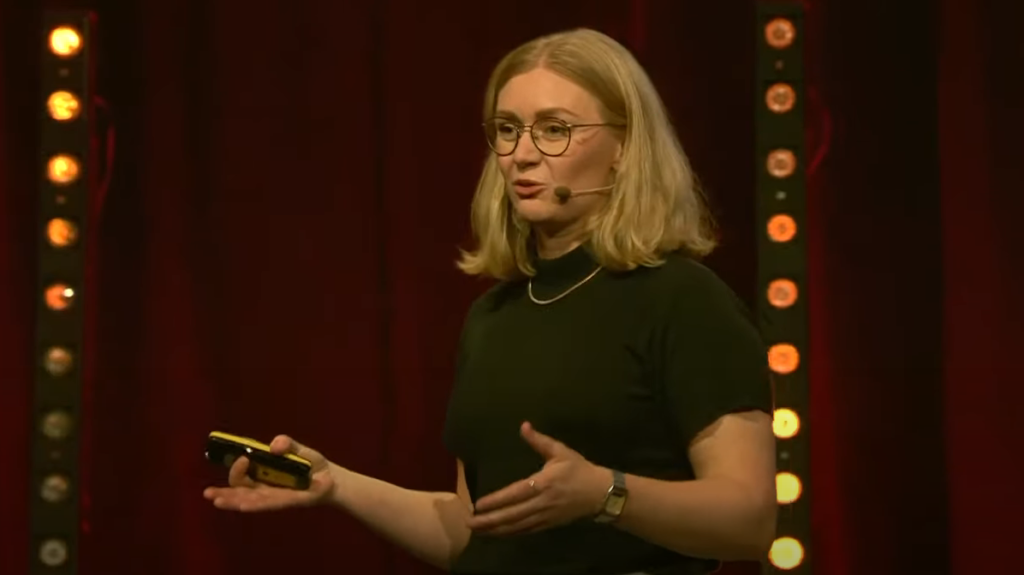
Vilde Bråten has submitted the following academic thesis as part of the doctoral work at the Norwegian University of Science (NTNU):
“Fundamental Aspects of Thermodynamics of Small Systems Investigated Through Molecular Simulations and Theoretical Descriptions”
Assessment Committee
The Faculty of Natural Science has appointed the following Assessment Committee to assess the thesis:
- Associate Professor, PhD Christelle Miqueu, Univ. Pau & Pays Adour, LFCR (UMR 5150), France
- PhD Ivan Latella, Laboratoire Charles Fabry, Institut d`Optique, France
- Professor Astrid de Wijn, Department of Mechanical and Industrial Engineering, NTNU
Professor Astrid de Wijn has been appointed Administrator of the Committee. The Committee recommends that the thesis is worthy of being publicly defended for the PhD degree.
Supervisors
The doctoral work has been carried out at the Department of Materials Science and Engineering, where Associate Professor Sondre Kvalvåg Schnell has been the candidate’s supervisor. Professor Øivind Wilhelmsen, Department of Chemistry has been the candidate’s co-supervisor.
Public trial lecture:
Time: 30 September 2022 at 10.15
Place: Disputasrommet, Main Building, NTNU Gløshaugen
Prescribed subject: “The relevance of thermal diffusion in practical applications”
Public defence of the thesis:
Time: 30 September 2022 at 13.15
Place: Disputasrommet, Main Building, NTNU Gløshaugen
You can also follow the trial lecture and defence via Zoom:
https://NTNU.zoom.us/j/92330824577?pwd=NjVWa2lRREFQK1Q3TGVBMXV4TWFDZz09
Passcode: 638663
Summary
Systems on the nanoscale can show behavior that is strikingly different from the behavior we expect for systems on the macroscopic scale. As a consequence, we can in general not assume that macroscopic theories of matter can be used to describe the properties of small systems. Thermodynamics was initially derived for systems with number of molecules on the order of Avogadro’s number. In this work, we explore the elements needed to apply thermodynamics to small systems, containing a much smaller number of molecules. We utilize molecular simulations combined with theoretical descriptions for direct investigations of the systems. We focus on three central questions: (1) How are thermodynamic properties affected by system size? (2) Can we define a consistent thermodynamic framework for small systems? (3) Which computational tools and techniques can be applied to study small systems?
For the first question, we investigate two features of small systems: size- and shape-dependent properties and ensemble in-equivalence. We show how thermodynamic properties can be conveniently described by scaling laws, and discuss the implications of ensemble in-equivalence. For the second question, we compare two theoretical descriptions that provide thermodynamic frameworks for small systems: Gibbs’ surface thermodynamics and Hill’s nanothermodynamics. We discuss the advantages and limitations of both methods, and give examples of systems where one framework is favored over the other. For the third question, we show how some statistical mechanical identities derived for macroscopic systems do not provide accurate predictions for systems with a small number of molecules. We also discuss some tools and techniques that can be trusted to provide accurate predictions of properties for small systems.
In Article I, we present a new method for computation of chemical potential differences. The method is based on a sampling technique that computes grand canonical particle fluctuations from sub-systems embedded in a larger reservoir. This sampling technique is combined with an overlapping distribution method that extracts chemical potential differences from the overlap between distributions of particle numbers from two systems at different densities. The small sub-systems are non-periodic, which means that their properties deviate from the classical thermodynamic description. We therefore utilize scaling laws to calculate the size dependence of chemical potential differences and eventually extract the value in the thermodynamic limit. In addition to computing chemical potential differences in the macroscopic limit directly from molecular dynamics simulation, the new method allows for investigation of the size dependence of intensive thermodynamic properties of small systems.
In Article II, we investigate how a small number of particles can induce an ensemble dependence to the properties of grand canonical (open) and canonical (closed) systems. We investigate cubic boxes with a surface energy, containing ideal gases or particles interacting through interatomic potentials. For all the investigated small systems, we find clear differences between the properties in open and closed systems. Through analytical derivation, we find that the properties of the ideal gases are affected by two types of small-size effects. The first type arises from the surfaces, edges and corners of the system, while the second type arises from avoiding assumptions about the magnitude of the number of particles. We utilize the insight gained from investigating the ideal gases to analyze ensemble dependence of systems with interacting particles. For the systems with interacting particles, the difference in chemical potential is qualitatively described by the analytic formula derived for the ideal gas system, while the difference in pressure is not captured by the ideal gas model. The work presented in this paper gives insight into the mechanisms behind ensemble in-equivalence in small systems, and illustrates how simple statistical mechanical models can provide useful information.
In Article III, we present a thermodynamic framework that represents an equation of state for pure fluids confined in small geometries. The total system consists of a bulk phase in equilibrium with a surface phase. We base the equation of state on an existing, accurate description of the bulk fluid, and use Gibbs’ framework for surface excess properties to consistently incorporate contributions from the surface. We demonstrate the equation of state for a Lennard-Jones spline fluid confined by a spherical surface with a Weeks-Chandler-Andersen wall-potential. The pressure and internal energy predicted from the equation of state are nearly within the accuracy of the properties computed directly from molecular dynamics simulations. We also investigate how the properties of the surface phase depend on the location of the dividing surface, and find that when the location is chosen appropriately, the properties of highly curved surfaces can be predicted from those of a planar surface. A possible application of the equation of state for confined fluids is prediction of thermodynamic properties in porous media.
Populærvitenskapelig sammendrag
Nanosystem er små samlinger av atomer som til sammen ikke er større enn en hundretusendel av et sandkorn. Disse små systemene oppfører seg veldig annerledes enn store system. Dette skjer fordi molekyler i nærheten av en overflate oppfører seg annerledes enn molekylene i midten av systemet, i den delen vi kaller bulken. I store system kan vi ofte se bort ifra bidraget til molekylene i nærheten av overflaten siden de utgjør en så liten andel av det totale systemet. Mens i små system befinner mange av molekylene seg ved overflaten til systemet, som gjør at de i mye større grad bidrar til hvordan systemet oppfører seg.
De helt spesielle egenskapene til små system åpner en ny dimensjon av muligheter for utvikling av teknologi. Nanoteknologi brukes for eksempel til å lage materialer med stort overflateareal, slik som porøse materialer som brukes i solceller, batterier og rensing av vann. Vi kan også spesialdesigne små nanopartikler med spesifikke egenskaper. Nanopartikler av gull kan blant annet brukes som katalysatorer, som får reaksjoner til å gå raskere, mens gull i bulk-form har ingen katalysatoregenskaper. Nanopartikler har også en helt unik evne til å passere gjennom biologiske barrierer i kroppen. De kan brukes til å transportere og levere medisin inni kroppen på en mer målrettet måte vi gjør i dag.
Gjennom arbeidet med denne doktoravhandlingen har vi jobbet med å forstå små system bedre ved å undersøke de gjennom simuleringer. Simuleringene regner ut hvordan atomene inni nanosystemet oppfører seg. Det gjør at vi kan se forskjellen mellom molekylene som er i bulken og de som er i nærheten av overflaten. På den måten kan vi få svar på hvordan og hvorfor små system oppfører seg sånn som de gjør, slik at vi kan utnytte de spennende egenskapene i fremtidens teknologi.